Research
Our lab is based in the Institute of Applied Physics and Materials Engineering, University of Macau. We are interested in the intriguing fundamental science emerged upon imposing strong confinement of nanometer down to angstrom in size. The research activities in this lab are highly interdisciplinary and closely related to 2D materials, nanofluidics and molecular sieving. The key knowledge learnt during the advanture is expected to lead to new technologies in environment (separation), informatices (biomimetic signaling and communication), medical (diagnostics) and energy (blue energy harvesting).
Representative works
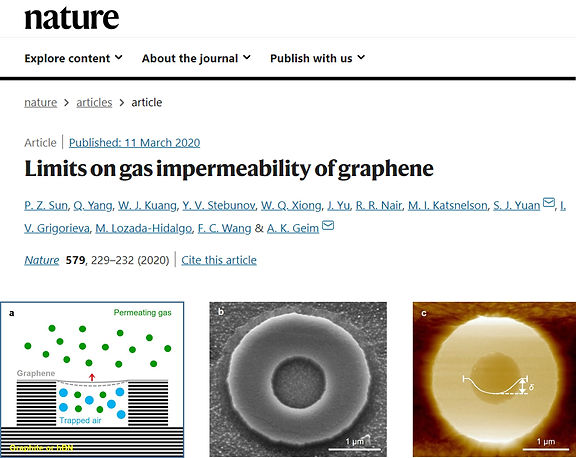
Despite being only one-atom thick, defect-free graphene is considered to be completely impermeable to all gases and liquids. This conclusion is based on theory and supported by experiments that could not detect gas permeation through micrometre-size membranes within a detection limit of 10^5 to 10^6 atoms per second. Here, using small monocrystalline containers tightly sealed with graphene, we show that defect-free graphene is impermeable with an accuracy of eight to nine orders of magnitude higher than in the previous experiments. We are capable of discerning (but did not observe) permeation of just a few helium atoms per hour, and this detection limit is also valid for all other gases tested (neon, nitrogen, oxygen, argon, krypton and xenon), except for hydrogen. Hydrogen shows noticeable permeation, even though its molecule is larger than helium and should experience a higher energy barrier. This puzzling observation is attributed to a two-stage process that involves dissociation of molecular hydrogen at catalytically active graphene ripples, followed by adsorbed atoms flipping to the other side of the graphene sheet with a relatively low activation energy of about 1.0 electronvolt, a value close to that previously reported for proton transport. Our work provides a key reference for the impermeability of two-dimensional materials and is important from a fundamental perspective and for their potential applications.
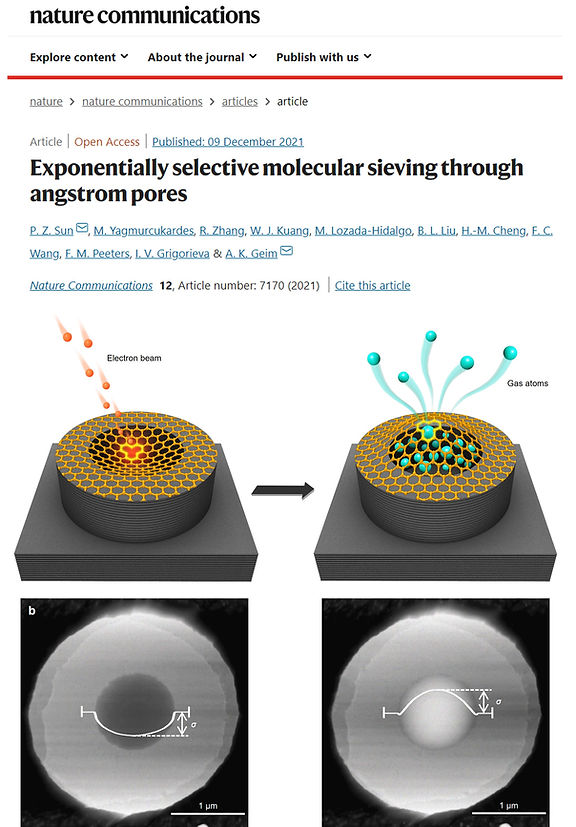
Two-dimensional crystals with angstrom-scale pores are widely considered as candidates for a next generation of molecular separation technologies aiming to provide extreme, exponentially large selectivity combined with high flow rates. No such pores have been demonstrated experimentally. Here we study gas transport through individual graphene pores created by low intensity exposure to low kV electrons. Helium and hydrogen permeate easily through these pores whereas larger species such as xenon and methane are practically blocked. Permeating gases experience activation barriers that increase quadratically with molecules’ kinetic diameter, and the effective diameter of the created pores is estimated as ∼2 angstroms, about one missing carbon ring. Our work reveals stringent conditions for achieving the long sought-after exponential selectivity using porous two-dimensional membranes and suggests limits on their possible performance.

Graphite is one of the most chemically inert materials. Its elementary constituent, monolayer graphene, is generally expected to inherit most of the parent material’s properties including chemical inertness. Here, we show that, unlike graphite, defect-free monolayer graphene exhibits a strong activity with respect to splitting molecular hydrogen, which is comparable to that of metallic and other known catalysts for this reaction. We attribute the unexpected catalytic activity to surface corrugations (nanoscale ripples), a conclusion supported by theory. Nanoripples are likely to play a role in other chemical reactions involving graphene and, because nanorippling is inherent to atomically thin crystals, can be important for two-dimensional (2D) materials in general.
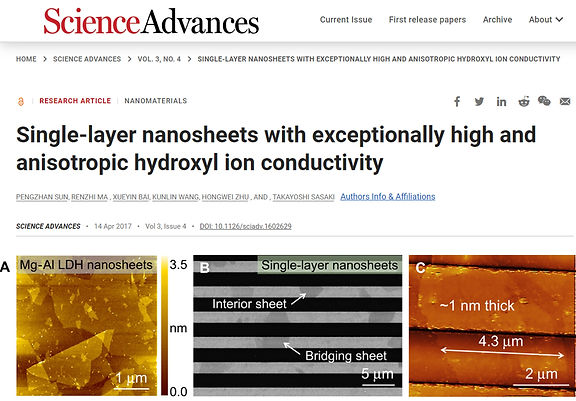
When the dimensionality of layered materials is reduced to the physical limit, an ultimate two-dimensional (2D) anisotropy and/or confinement effect may bring about extraordinary physical and chemical properties. Layered double hydroxides (LDHs), bearing abundant hydroxyl groups covalently bonded within 2D host layers, have been proposed as inorganic anion conductors. However, typical hydroxyl ion conductivities for bulk or lamellar LDHs, generally up to 10^−3 S per cm, are considered not high enough for practical applications. We show that single-layer LDH nanosheets exhibited exceptionally high in-plane conductivities approaching 10^−1 S per cm, which were the highest among anion conductors and comparable to proton conductivities in commercial proton exchange membranes (for example, Nafion). The in-plane conductivities were four to five orders of magnitude higher than the cross-plane or cross-membrane values of restacked LDH nanosheets. This 2D superionic transport characteristic might have great promises in a variety of applications including alkaline fuel cells and water electrolysis.

Two-dimensional (2D) materials offer a prospect of membranes that combine negligible gas permeability with high proton conductivity and could outperform the existing proton exchange membranes used in various applications including fuel cells. Graphene oxide (GO), a well-known 2D material, facilitates rapid proton transport along its basal plane but proton conductivity across it remains unknown. It is also often presumed that individual GO monolayers contain a large density of nanoscale pinholes that lead to considerable gas leakage across the GO basal plane. Here we show that relatively large, micrometer-scale areas of monolayer GO are impermeable to gases, including helium, while exhibiting proton conductivity through the basal plane which is nearly two orders of magnitude higher than that of graphene. These findings provide insights into the key properties of GO and demonstrate that chemical functionalization of 2D crystals can be utilized to enhance their proton transparency without compromising gas impermeability.